Engine Development Division
Industrial Power Products Management Division
Power Solution Business
YANMAR Technical Review
Prediction for SCR Systems Performance using 3D CFD Simulation: Aiming at SCR Development for Various Layouts
Abstract
Selective catalytic reduction (SCR) system is an effective diesel aftertreatment technology for complying with stringent nitrogen oxides (NOx) emission regulations. SCR systems need to maximize NOx reduction and minimize the formation of deposits, which cause problems for the engine and SCR system. Both are significantly influenced by how the urea water solution (UWS) is injected and vaporized, and by the diffusion of ammonia in the exhaust gases. This makes mixing pipe design one of the most important aspects of an SCR system.
The Yanmar diesel engines used in industrial machinery require a variety of different SCR system layouts. Yanmar used a 3D CFD simulation developed for its own equipment and with reference to the literature to create a design technique that can optimize the mixing pipe in new SCR layouts. Experimental testing demonstrated good agreement between the 3D CFD simulation results and both ammonia distribution measured at the inlet surface of the SCR catalyst and deposit formation.
1. Introduction
Diesel engines are widely used in industrial machinery because of their fuel efficiency and high levels of reliability and durability. However, emissions regulations for these industrial diesel engines are becoming progressively tighter in recognition of the effect on the environment of the nitrogen oxides (NOx) and particulate matter (PM) contained in their exhaust gases. In developed countries such as Japan, Europe, and the United States, industrial diesel engines of 56kW or more are being required to significantly reduce their NOx emissions compared to the levels permitted in past regulations. In the case of Yanmar's 4TNV94FHT engine, for example, the US Tier 4 Final regulations mandate NOx emissions to be reduced to one-tenth the level permitted in the previous Tier 4 Interim regulations. As a result, most industrial diesel engines of 56kW or more are being fitted with selective catalytic reduction (SCR) systems using urea water solution (UWS) to reduce NOx and thereby comply with the emission regulations.
SCR systems spray UWS into the exhaust pipe to form ammonia (NH3), which then reduces the NOx in the exhaust gas. The keys to achieving a high level of NOx reduction together with reliable operation lie in the behavior of the UWS droplets, their evaporation, and the dispersion of NH3 through the exhaust gas. These are strongly influenced by the shape of the mixing pipe and the positioning of the injector. However, because the exhaust systems of industrial machinery have a variety of different layouts, there is a need in SCR system development for mixing pipe design techniques that can take account of the SCR layout.
This article describes a technique for the analysis and prediction of SCR system performance developed to improve mixing pipe design practices.
2. Overview of SCR System
2.1. NOx Reduction Mechanism in SCR Systems
The SCR system is made up of an injector that sprays UWS into the pipe, a mixing pipe in which the sprayed UWS is converted into NH3 and NH3 is dispersed into the exhaust gas, and a case containing the SCR catalyst.
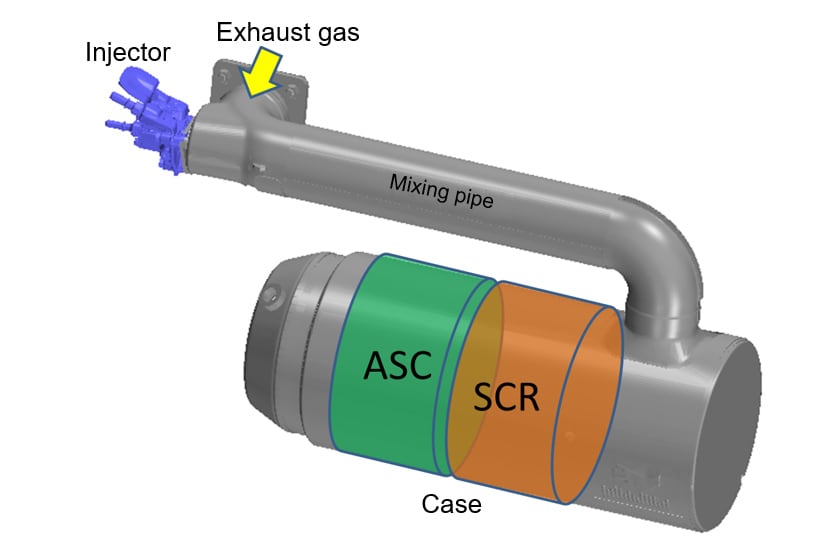
Fig. 2 shows the mechanism by which the SCR system reduces NOx. The UWS droplets sprayed from the injector impinge on the walls and are either broken up or adhere to the wall surface before vaporizing. They are then converted to NH3 by either thermolysis (1) and hydrolysis (2) as they mix with the exhaust gas before reaching the SCR catalyst. The NH3 and NOx then are reacted and broken down to nitrogen (N2) and water (H2O) on the catalyst (3).
Thermolysis: (NH2)2CO → NH3 + HNCO (1)
Hydrolysis: HNCO + H2O → NH3 + CO2 (2)
Reaction on SCR Catalyst: 2NH3 + NO + NO2 → 2N2 + 3H2O (3)
If the amount of NH3 supplied is insufficient for the NOx in the exhaust gas, NOx "slips" through the SCR catalyst unreacted. Similarly, any excess of NH3 relative to NOx also "slips" through unreacted. The term "slip" is used to refer to this condition of passing through the SCR catalyst unreacted. NH3 slip is converted to nitrogen, water, and NOx by the ammonia slip catalyst (ASC) located downstream of the SCR catalyst, and then exhausted into the atmosphere. This means that an excess or insufficiency of NH3 relative to NOx will potentially result in a failure to comply with emission regulations.

2.2. Design Issues for SCR Systems
Along with providing a high performance for NOx reduction, SCR systems also need to minimize the formation of deposits in the mixing pipe.
The design of the mixing pipe is an extremely important part of SCR system development, because NOx reduction performance and deposits are strongly influenced by the behavior and evaporation of UWS droplets and dispersion of NH3 in the mixing pipe.
(1) NOx reduction performance
If the layout provides only a short distance between the injector and SCR catalyst, or if a mixing pipe with enough length is used but urea evaporation or NH3 mixing is insufficient, wide variations in the concentration of NH3 in the exhaust gas occur at the inlet surface of the SCR catalyst. As regions of high NH3 concentration have an excess of NH3 over NOx, NH3 slips through the SCR catalyst in these places. Similarly, regions of low NH3 concentration have an insufficiency of NH3 relative to NOx, so in these places NOx slips the SCR catalyst. This means that NOx reduction performance is strongly influenced by how well NH3 mixing is achieved.
(2) Deposits
Table 1 lists the main components of deposits and the conditions under which they form. It is known that deposits can form when urea fails to vaporize and instead remains as a liquid film on the mixing pipe, or when circulation of the exhaust gas flow results in a high concentration of isocyanic acid (HNCO) formed by the thermolysis of urea(1)(2).
Table 1 Deposit Composition and Conditions for Formation

Deposits formed in the vicinity of the injector block the UWS spray, degrading NOx reduction or causing problems in the injector(3). Similarly, the formation of deposits in the exhaust gas flow path not only degrades NOx reduction by getting in the way of the UWS droplets and impeding NH3 dispersion in the mixing pipe, it can also result in engine problems due to the higher exhaust back pressure, or damage to the SCR catalyst due to the impact of deposits that break off from the walls(4)(5).
While the main deposit components listed in Table 1 decompose at temperatures of 300°C or less, other compounds that form when these components react together, such as ammelide, melem, or melon, do not break down at high temperatures and there is a risk that these deposits will remain permanently on the mixing pipe or the SCR catalyst case(6).

3. Numerical Analysis of SCR System
3.1. Development of Analysis Model
SCR systems are required to have a variety of different layouts to allow for installation on different machinery, with a new mixing pipe design being required each time a new layout is developed. Because it is not possible experimentally to observe things like the behavior of the UWS spray in the pipe or the deposit formation process, an optimal pipe design based on experiments is difficult. Moreover, the prototyping and experimental performance testing of mixing pipes takes a lot of time and work. Accordingly, computational fluid dynamics (CFD) provides a useful way to design and assess mixing pipes when developing a new layout.
To analyze SCR system performance and express the phenomena happening inside the mixing pipe, it is necessary to model all the processes from spraying the UWS into the exhaust pipe until it reaches the SCR catalyst in the form of NH3. An analysis model was developed with reference to research results by Birkhold, et al.(7) for the evaporation characteristics of UWS, Smith, et al.(9) for spray impingement on walls, and Fischer, et al.(8) and Zöchbauer, et al.(10) for mixing of NH3 in the exhaust gas. It is known that, among these factors, the model for spray impingement on the wall has an especially strong influence on NOx reduction performance and deposits formation(9)(11). Fig. 4 shows the UWS droplet behaviors after impinging on a wall depending on the wall temperature and the Weber (We) number of the impinging droplets(12).
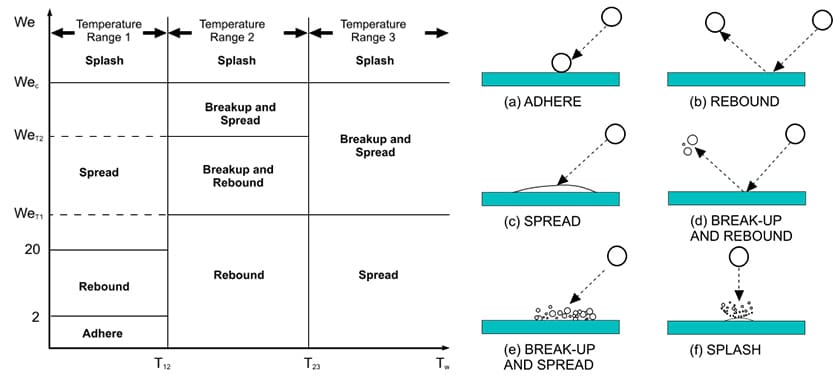
While the risk of deposit formation is low in rebound mode, for example, because the droplets do not remain as a liquid film after impinging on the wall, the droplets reach the SCR catalyst without having broken up and this tends to result in less uniformity of NH3 at the inlet surface of the SCR catalyst. In spread mode, the droplets form a liquid film on the wall after impingement and therefore evaporation of the UWS takes place on the wall. Although this provides adequate NH3 mixing distance in the mixing pipe and therefore tends to result in higher uniformity in the concentration of NH3 at the inlet surface of the SCR catalyst, it is also a cause of deposit formation from the liquid film. As the behavior of droplets when they impinge on the wall also depends on factors such as the surface roughness of the wall, specific experiments were also conducted on the mixing pipe used in the actual SCR system to improve the accuracy of the model for spray impingement on the wall.
3.2. Analysis Method
(1) NH3 uniformity
If the theoretical amount of NH3 is supplied relative to the NOx in the exhaust gas and the reaction rate at the SCR catalyst is fast enough, the NOx reduction rate is equal to the NH3 uniformity defined in equation (4)(13). Accordingly, the analysis uses NH3 uniformity to determine the variation in the concentration of NH3 at the inlet surface of the SCR catalyst shown in Fig. 5.
Although gaseous urea or HCNO is present at the inlet surface of the SCR catalyst if the thermolysis or hydrolysis of the urea is insufficient, it is known that these are rapidly converted to NH3 inside the SCR catalyst. Therefore, the calculation of NH3 uniformity at the inlet surface of the SCR catalyst assumes that the gaseous urea and HCNO are converted to NH3.

(2) Deposits
As described in section 2, the deposits listed in Table 1 occur when a film of liquid urea forms on the walls or there is a high concentration of HNCO in the exhaust gas, and the temperature at the corresponding point on the wall satisfies the conditions. This makes it a proper idea to use wall temperature as the criterion for making an accurate determination of whether or not deposits form under given conditions(1)(2). However, because the wall temperature is influenced by the ambient air temperature, there is a risk that including wall temperature in the criteria will not always produce proper conclusions when performing a comprehensive evaluation of the mixing pipe under a variety of different machine operating conditions. For this reason, the film of liquid urea, HNCO concentration, and circulation in the exhaust gas flow were chosen as the criteria for evaluating the risk of deposit formation in the analysis.

3.3. Results of Numerical Analysis
(1) NH3 uniformity analysis
To verify the accuracy of the analysis, the distribution of NH3 at the inlet surface of the SCR catalyst was measured for two different layouts of the mixing pipe shown in Fig. 6 (mixing pipe A and mixing pipe B) each of which had a different internal design. As with the analysis, the results for NH3 uniformity at the inlet surface of the SCR catalyst in the experiment was obtained with converting HNCO and gaseous urea to the equivalent amount of NH3. Good agreements between analysis and experiment were obtained for the NH3 distribution of both layouts, demonstrating the validity of the NH3 uniformity analysis.
Table 2 Conditions for NH3 Distribution Experiment and Analysis


(2) Deposit analysis
To verify the accuracy of the deposit analysis, experiments and analysis were conducted under two operating conditions (O.P.1 and O.P.2) for the mixing pipe shown in Fig. 7. The analysis results indicated no flow circulation in exhaust gas and a low concentration of HNCO for both conditions. Fig. 7 shows the analysis results of liquid film thickness distribution and pictures of deposits by the experiments. The deposit formation obtained by the experiments was in good agreement with the presence or not of liquid urea film indicated by the analysis, demonstrating the validity of the deposit analysis.
Table 3 Conditions for Deposit Experiment and Analysis


3.4. Cases Study of Using Analysis to Improve Deposits
This section describes an example of the analysis used to improve the mixing pipe. Deposits were observed on the inside of the curved original mixing pipe on which an injector is attached as shown in Fig. 8. When the analysis was used to make improvements, the results indicated that deposit formation could be reduced by changing the attaching angle of the injector. Experimental testing of a mixing pipe with this new injector attaching angle confirmed that deposit formation was in fact reduced.
Table 4 Conditions for Deposit Experiment and Analysis


4. Conclusions
The development of a numerical analysis technique for evaluating SCR system mixing pipes obtained the following results.
- NH3 uniformity, which contributes NOx reduction rate, was predicted analytically and the validity was verified by experiments.
- Deposit formation was predicted analytically and the validity was verified by experiments. The analysis was also used to make improvements to the mixing pipe and the effect of controlling deposit formation was confirmed by experiments.
Along with the mixing pipe design technique described in this article, SCR systems also require a variety of other technologies such as SCR catalyst selection and UWS spray control. Yanmar intends to continue making technical improvements to SCR systems so as to satisfy diverse customer requirements for incorporating SCR.
5. References
- (1)Smith, H., et al., "Optical and Numerical Investigations on the Mechanism of Deposit Formation in SCR Systems", SAE Technical Paper 2014-01-1563, 2014, doi:10.4271/2014-01-1563.
- (2)Smith, H., et al., "Evaluation and Prediction of Deposit Severity in SCR Systems", SAE Technical Paper 2016-01-0970, 2016, doi:10.4271/2016-01-0970
- (3)Munnannur, A., et al., "Thermal and Fluid Dynamic Considerations in Aftertreatment System Design for SCR Solid Deposit Mitigation", SAE Technical Paper 2012-01-1287, 2012, doi:10.4271/2012-01-1287.
- (4)Kornell De Rudder "Tier 4 High Efficiency SCR for Agricultural Applications", SAE Technical Paper 2012-01-1087, 2012, doi:10.4271/2012-01-1087.
- (5)Xu, L., et al., "Laboratory and Engine Study of Urea-Related Deposits in Diesel Urea-SCR After-Treatment Systems", SAE Technical Paper 2007-01-1582, 2007, doi:10.4271/2007-01-1582.
- (6)Colin L. Weeks, et al., "Investigation of Urea Deposit in SCR System", SAE Technical Paper 2015-01-1037 doi:10.4271/2015-01-1037
- (7)Birkhold, F., et al., "Analysis of the Injection of Urea-Water-Solution for Automotive SCR DeNOx-Systems: Modeling of Two-Phase Flow and Spray/Wall-Interaction", SAE Technical Paper 2006-01-0643, 2006, doi:10.4271/2006-01-0643.
- (8)Fischer, S., et al., "Impact of the Turbulence Model and Numerical Approach on the Prediction of the Ammonia Homogenization in an Automotive SCR System", SAE Int. J. Engines 5(3):1443-1458, 2012, doi:10.4271/2012-01-1291.
- (9)Smith, H., et al., "Advanced Spray Impingement Modelling for an Improved Prediction Accuracy of the Ammonia Homogenization in SCR Systems", SAE Technical Paper 2015-01-1054, 2015, doi:10.4271/2015-01-1054.
- (10)Zöchbauer, M., et al. "Advanced SCR Flow Modeling with a validated Large Eddy Simulation", SAE Technical Paper 2015-01-1046, 2015, doi:10.4271/2015-01-1046.
- (11)Bhattacharjee, S., et al. "CFD Modeling of Processes Upstream of the Catalyst for Urea SCR NOx Reduction Systems in Heavy-Duty Diesel Applications", SAE Technical Paper 2011-01-1322, 2011, doi:10.4271/2011-01-1322.
- (12)Bai, C., et al. "Development of Methodology for Spray Impingement Simulation", SAE Technical Paper 950283, 1995, doi: 10.4271/950283.
- (13)CD-adapco, Users Manual Version 11.02, 2016.
- (14)Mckinley, T., et al. "Mixture Non-Uniformity in SCR Systems: Modeling and Uniformity Index Requirements for Steady-State and Transient Operation", SAE Technical Paper 2010-01-0833, 2010, doi:10.4271/2010-01-0883.
-IMPORTANT-
The original technical report is written in Japanese.
This document was translated by Research & Development Management Division.
Author
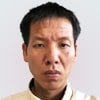